Boyang (Bobby) Zhang
Do lanthanum (La) and actinium (Ac) or lutetium (Lu) and lawrencium (Lr) belong to group 3 under scandium (Sc) and yttrium (Y), along with the d-block elements, in the periodic table?
Abstract
More than three decades after William Jensen’s article initiated the group 3 debate of the periodic table in 1982, the chemistry community has not yet reached a consensus towards whether lanthanum (La) and actinium (Ac) or lutetium (Lu) and lawrencium (Lr) should be placed in group 3 under scandium (Sc) and yttrium (Y). It is desirable to have a recommended version of the periodic table since multiple versions of periodic tables can be confusing for many people. Though there is specific evidence that validates the placement of lanthanum and actinium, and though it is important to consider chemical properties, such an approach is limited in scope, leading to conflicting conclusions. Recently, more conclusive arguments that promote the Lu, Lr arrangement can resolve this issue by approaching it from a global perspective, emphasizing that the periodic table should be organized strictly by overall principles.
The iconic periodic table created over two centuries ago has recently fallen into an awkward situation — it has been questioned whether it assigns every element to its rightful place. The elements in group 3 start with scandium (Sc) and yttrium (Y), but what are the next two elements? Periodic tables across textbooks, posters, and websites may offer varied answers to this question. After element 56, barium (Ba), there is an interruption between the s-block and d-block; here, one navigates to the f-block annex — also known as the lanthanoids (lanthanides) and actinoids (actinides) — under the main body. The lanthanoids and actinoids series often fall under Sc and Y, but what exact elements should belong to the same group as Sc and Y have been at the center of debate — hence the group 3 dilemma. One side of the debate puts the first two elements of the f-block, lanthanum (La) and actinium (Ac), under group 3, while the other side chooses the last two, lutetium (Lu) and lawrencium (Lr), in the block to be in group 31. The debate has slowly gained momentum over four decades. Advocates for either choice of placement have proposed various evidence to support their claim; however, the chemistry community has not yet reached an agreement to date. Though there is specific evidence that validates the placement of lanthanum and actinium, and though it is important to value chemical properties considering that is how Mendeleev first navigated periodicity, such an approach is limited in scope — leading to conflicting conclusions that are valid for both choices. Recently, more conclusive arguments that promote the Lu, Lr arrangement can resolve the exhaustive debate by applying a global perspective, emphasizing that the periodic table should be organized strictly by overall trends and principles.
Admittedly, the Sc, Y, La, Ac arrangement has a stronger historical basis; advocates for La and Ac believe that elements should be arranged based on how they react, and such belief has historical basis traced back to when the periodicity was first created. There is evidence that lanthanum does behave in more chemically similar ways to Sc and Y than lutetium does. In a study focusing on how binary compounds can inform periodic trends, Restrepo and colleagues compare over 4,700 diatomic compounds from the combination of 94 elements across the periodic table, including some lanthanides and actinides. Specifically, two elements are deemed to be chemically similar and have relational properties when they can form binary compounds with a third element. Based on the similarity landscape mapped by the study, there are more cases that La forms more similar compounds with Sc and Y than Lu does, therefore suggesting that La behaves more similar to the group 3 elements in chemical reactions and has a more rightful place in group 3. In an interview with C&EN, the study’s primary investigator Restrepo further strengthens the La, Ac argument by alluding the connection to a historical fact. He believes that behavior and reactivity should be the deciding principle in arranging the element since, historically, forerunners such as Mendeleev studied periodicity from the same approach — by researching how elements interact with each other. As stated by Restrepo, “at the core of periodic system, all you have is chemistry” when continuing building on the work of famous precedents.
Arguments in favor of La and Ac contend to retain this principle in which specific chemical properties and similarities are prioritized. Upon close scrutinization of the elements in question for their eligibility to be put together with the d-block elements under Sc and Y, the supporters for La, Ac placement presents some reasonable explanations about the electron configurations that Lu, Lr, in comparison, would be a less favored fit. Laurence Lavelle, a faculty member of the Department of Chemistry and Biochemistry at UCLA, acutely points out that it would make no sense to keep La and Ac in the f-block regarding their valence electron configurations. For lanthanum ([Xe]5d16s2) and actinium ([Rn]6d17s2), both elements have no electrons in the f-orbital, and instead, they have one electron in the d-orbital. As a result, it would be illogical to call them f-block elements when they have no electron in that orbital at all, and Lavelle argues it can be confusing, especially for students who are learning electron configurations.
Admittedly, this argument is immensely effective in supporting the candidacies of La and Ac, since Lu and Lr, on the other hand, have a fully filled f-orbital outside their respective noble gas cores. Nonetheless, there is equally valid proof promoting the placement of Lu and Lr on the other end of the debate. William B. Jensen’s influential 1982 article published in the Journal of Chemical Education is generally agreed upon to be the earliest in the field who addressed the group 3 debate. Contrary to the electron configuration argument proposed by Lavelle, Jensen offers convincing explanations of why La and Ac should indeed be considered f-block elements. He underlines that the d-orbital electrons of La and Ac are merely irregularities. The neighboring element, thorium (Th, [Rn]6d27s2), is of a similar case and is widely considered as an anomaly, whereas all eighteen other f-block elements conform to the idealized (n – 2)f1ns2 configuration (1982, p. 635). Consequently, the f-block should start with La and Ac as the first members and end with ytterbium (Yb) and nobelium (No), and the d-block elements in periods 6 and 7 should start with Lu and Lr. In this way, it brings an extra level of harmony that all d-block elements would all have a consistent [noble gas](n – 2)f14 core.
As the counterevidence for Restrepo’s binary compound study, Jensen also showcases better intragroup analogies in the particular physical and chemical properties between Lu, Lr, and d-block elements. He gathered data from the works of other physicists and chemists at the time and presents the periodic trends in two tables based on various properties such as atomic radius, melting point, and electronegativity (1982, p. 635-36). Results show that Lu better conforms to the intragroup trends than La does; Lu also shares many properties — such as highest oxidation state, structure of oxide, and superconductivity — with Sc and Y, while these correlations are absent in La’s case. As a result, Jensen offers some solid validations as to why the Lu, Lr placement is an equally qualified consideration — if not better — as the La, Ac placement in the d-block.
From 2008 to 2015, multiple rounds of heated exchange between Jensen and Lavelle have drawn an increasing amount of attention to the debate. In 2015, IUPAC initiated a project to evaluate all evidence in order to eventually offer an official recommendation on which elements belong to group 3; however, five years later, the task group has not reached any conclusion. It seems that the group 3 dilemma has come to an impasse.
However, in 2018, Eric Scerri and William Parsons propose a refreshing approach to the group 3 issue that may resolve the stalemate. This new argument emerged to conclude that solely focusing on specific chemical and physical properties of the elements can only lead to conflicting and inconclusive arguments. It should be pointed out that most arguments endeavored by both ends of the dispute to date are focused on the atomic composition, specific properties, or local trends. In this book chapter, Scerri and Parsons describe the particular chemical and physical data, which these arguments build upon, is inconclusive in nature (p. 145). For instance, both Lavelle’s and Jensen’s arguments about electron configurations are partially effective in supporting their cases and disproving their respective opponent’s, but such evidence does not help to reach an overall conclusion. Moreover, Megan Fieser, an inorganic chemistry professor at USC, stresses that the group 3 issue is complex because there are many exceptions and irregularities all over the periodic table (personal communication, April 2, 2020). This further illustrates that the scope of reasoning should not be limited locally to specific evidence since it cannot be justified globally or applied extensively for other elements or periodic trends.
Instead, Scerri and Parsons suggest a global viewpoint emphasizing overall principles and patterns based on three “modest” principal rules. They believe that the periodic table should be organized strictly by theories, overall patterns, and global characteristics that encompass all members of the periodic table rather than dwelling on specific data or electron configurations of the elements concerned. The proposal is comprised of three reasonable, fundamental requirements that can be readily accepted by most chemists (p. 145-150). First, the common 18-column format is merely a pragmatic alternative based on the 32-column long-form that presents the full periodic table; that is to say, the arrangement of elements should make sense when presented in a long-form periodic table. Second, elements should always smoothly increase in atomic number in sequence from left to right. Lastly, the arrangement should adhere to the Aufbau principle, alternatively known as the Madelung Rule, which defines the order of orbital filling. Based on these principles, Lu and Lr naturally become the more appropriate candidates for group 3 as the placement meets all three conditions with no anomalies. On the other hand, incorporating La and Ac into group 3 would result in either visually awkward splitting the d-block into two highly uneven portions meanwhile violating the Madelung Rule, or an equally undesirable disruption in the increasing order of atomic number2. Therefore, this comprehensive approach succeeds in circumventing the pitfall of never-ending clashes of evidence and providing a categorical argument in favor of the placement of Lu and Lr.
In the end, while it is important to end the debate at the moment, it is of equal importance to raise awareness of the issue. An official ruling on the group 3 debate by IUPAC will significantly benefit a large crowd from physicists and chemists to instructors and students. In the classrooms, students may find different versions of the periodic table across textbooks puzzling, and the instructors might not be able to provide a good answer. This new model of reasoning hopefully saves instructors a few awkward moments and provides them with the confidence to give a clear-cut explanation that enables students to appreciate the beauty of the periodic table. For scientists, this will mark another milestone in organizing and refining a knowledge system for the elements. In this regard, adopting the new model that emphasizes global trends and builds on general principles resonates with the notion of science as the pursuit for general, systematic truths, and hence incorporating Lu and Lr to group 3 is a justified, favored choice that would better address the dilemma.
Select Bibliography
- Ball, P. (2017, April 21). The group 3 dilemma. Retrieved from https://www.chemistryworld.com/opinion/the-group-3-dilemma/3007080.article
- IUPAC. (2015, December 18). Project Details: The Constitution of Group 3 of the Periodic Table. Retrieved from https://iupac.org/projects/project-details/?project_nr=2015-039-2-200
- Jensen, W. B. (1982). The positions of lanthanum (actinium) and lutetium (lawrencium) in the periodic table, Journal of Chemical Education, 59(8), 634–636.
- Jensen, W. B. (2009). Misapplying the Periodic Law. Journal of Chemical Education, 86(10).
- Jensen, W. B. (2015). The positions of lanthanum (actinium) and lutetium (lawrencium) in the periodic table: an update. Foundations of Chemistry, 17(1), 23–31.
- Lavelle, L. (2008). Lanthanum (La) and Actinium (Ac) Should Remain in the d-block. Journal of Chemical Education, 85(11), 1482.
- Lavelle, L. (2008). Response to “The Flyleaf Periodic Table”. Journal of Chemical Education, 85(11), 1491.
- Lavelle, L. (2009). Response to Misapplying the Periodic Law. Journal of Chemical Education, 86(10).
- Lemonick, S. (2019, January 7). The periodic table is an icon. But chemists still can’t agree on how to arrange it. Chemical & Engineering News, 97(1). Retrieved from cen.acs.org/physical-chemistry/periodic-table/periodic-table-icon-chemists-still/97/i1.
- Restrepo, G. et al. (2012). A Network Study of Chemical Elements: From Binary Compounds to Chemical Trends. MATCH Communications in Mathamatical and in Computer Chemistry, 68, 417-442.
- Scerri, E. (2009). Which Elements Belong in Group 3? Journal of Chemical Education, 86(10), 1188.
- Scerri, E., & Parsons, W. (2018). What Elements Belong in Group 3 of the Periodic Table? In E. Scerri & G. Restrepo (Eds.), Mendeleev to Oganesson: A Multidisciplinary Perspective on the Periodic Table (pp. 140–151). New York, NY: Oxford University Press.
End Notes
1. The positions of lanthanum (La), actinium (Ac), lutetium (Lu), and lawrencium (Lr) are highlighted in the periodic table below (Retrieved from https://www.acs.org/content/acs/en/education/whatischemistry/periodictable.html). This periodic table avoids putting either La, Ac or Lu, Lr in group 3 by presenting a 15-column f-block. Many argue that although such arrangement circumvents the debate, it does not fundamentally solve the issue and can still cause confusion.
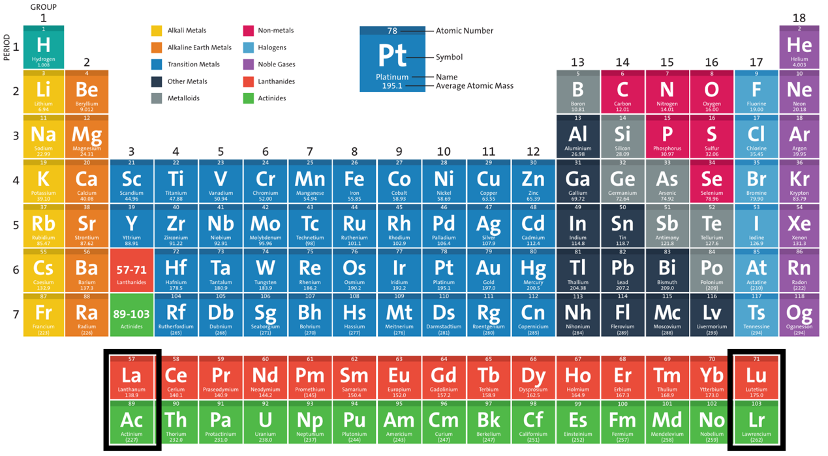
2. The figure below presents two versions of the long-form periodic table with Lu, Lr and La, Ac placement under Sc, Y. In this lower table, there are interruptions of the sequence of atomic number when navigating from Ba to Hf and Ra to Rf.
